Beginning to Develop Intuition for Energy¶
As an interesting port of departure, there was a BBC Horizons documentary called “Can We Make a Star on Earth? ” that came out in 2009 covering topics from nuclear fusion to world energy consumption. The show was hosted by British particle physicist Brian Cox, who adds a particular hip flair to all his projects, making for an enjoyable and informative exploration.
The reason to bring up the show, is it has two moments that are particularly intriguing. The first comes when Cox is interviewing energy consultant Saul Griffith on the topic of energy production/consumption cycles, and approaches to developing intuition for how these cycles work, as well as how they impact the cultures, economies, and people of our world. Initially in this scene, some numbers and statistics are tossed around, and those numbers, it turns out, provide an interesting backdrop by which to understanding the world’s energy production/consumption cycle.
The second scene of note, which comes chronologically later, is a meditative reflection on the nature of what is now frequently referred to as our global energy crisis. First let’s take a closer and more careful look at the argument presented in the first scene to see if we can outline the basic features at play within it, and to see if subsequently we can use it to develop a little intuition on how energy production and consumption cycles work.
At the outset it should be clearly stated that one of the basic goals here is that of simplification. We want to simplify the problem to the extent that we can start to develop a sense of how the basic features interact and move relative to each other. That said, we also want to avoid simplifying to a level where the analysis loses touch with any discernible reality (that is, loses touch with the world we actually live in), as seems to frequently be the case in television and internet ads designed by energy companies to distort the attributes of their products, and pervert the publics intuition.
In order to understand the basic movement of energy consumption/production, we need to have an understanding of the meaning of energy density and/or power density. Recall that power is simply the rate at which energy (or work) is performed. It turns out that with this concept alone, we can start to get a much sharper understanding of how energy consumption and production work. Some quotes from some prominent voices in energetics highlight the accepted importance of these relatively simple concepts.
“[Energy/]Power density
is perhaps the most revealing variable in energetics…”- Vaclav Smil
“It may be a bit of an exaggeration to say that understanding power[/energy] density may be all the average person requires to put our energy sources and needs into perspective, but there is some merit in this argument. Unfortunately, this view of energy matters remains little discussed, probably because it appears rather academic.” – Kent Hawkins
So what is energy/power density? The density of power or energy can be thought of as how much power (or energy per unit time, such as energy per second) can be produced per amount of substance used. For example, how much energy or power can be obtained from a single atom of uranium in nuclear fission, or from a single atom of water in a hydroelectric plant, or a single atom of air in a wind turbine, etc. The amount of substance can be measured in units of mass (such as how much power/energy per kilogram) or volume (such as how much energy per cubic meter or liter).
Why is energy and/or power density the right quantity for developing energy intuition? The simple reason is that in order to obtain energy or power from a given power resource in the real world, we must be able to actually access that given quantity. For example, if I want 16,000,000 Watts of continuous power for some reason, then that means that on average every second I need to extract 16,000,000 Joules of energy from my energy resource. If I know that wood has an energy density of 16 MJ/kg while falling water from a height of 100 meters (like in a hydroelectric dam) has an energy density of 0.001 MJ/kg, I know right away that one kilogram of wood has the same amount of energy as 16,000 kg of water falling 100 meters in a dam.
On its face then, energy or power density is a practical measure that provides a way of interpreting feasibility of energy resources. If the amount of energy I need requires more coal in terms of total mass than is currently present on or in the entire planet, then coal would not be a feasible solution for extracting the entirety of the energy I need.
It turns out that when looking closer at energy production/consumption dynamics, the power volume density can still be quite misleading. This is because power sources such as solar, wind, and hydroelectric generally require the construction of materials and technologies that are fastened to the surface of the earth. As a consequence, in these cases, the question often becomes: how much power or energy per unit surface area can be generated? It can be somewhat confusing, but this is still frequently referred to as power density, but instead of measuring in units of volume it is measured in units of area
. For a slightly more detailed discussion on this topic, links (1) and (2) provide a nice overview.
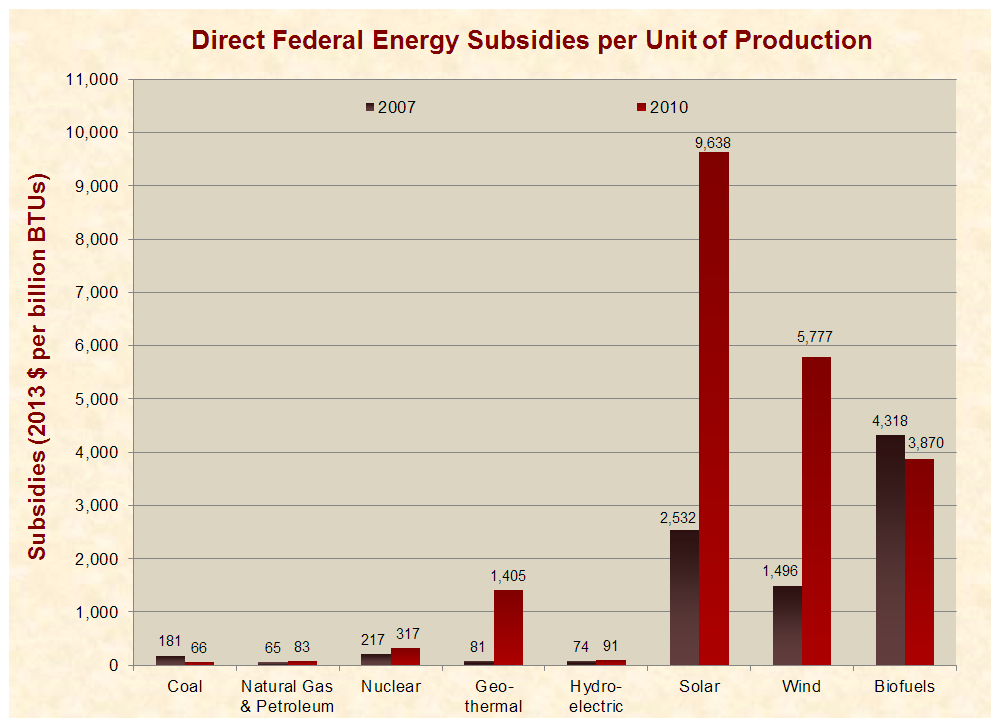
Of course, energy or power density is not the only important concern of any particular energy resource. Experts will quickly point out that, for example, efficiency, or roughly the amount of actual energy one can get from a resource, the types of byproducts produced (e.g. pollution, emission, etc. is created), the relative abundance of resource, its portability (ease of transport), and cost, are clearly important factors in determining the viability of any particular energy resource. Another interesting observation that we will revisit in much more detail later, is the fact that, somewhat remarkably, US government funding and subsidies for energy production research and development lies in almost direct opposition to the actual power density potential of a given energy resource.
We will discuss these additional aspects in more detail in subsequent entries, but at the outset it is important to recognize that though politicians and lobbying groups focus on many ancillary aspects of the energetics of specific energy resources, it turns out that they are surprisingly much less important to the most basic constraints introduced simply by understanding the effective energy and/or power density of a given resource. That is, effective energy and/or power density can be thought of as the most basic measure of what is possible or feasible in reality.
There are a number of additional cautionary comments. First, it will often be taken for granted that energy production is related to energy consumption directly. That is, the amount of energy consumed will be assumed to coincide directly in with the amount of energy produced. This is a convenient simplification, though should be taken with a grain of salt. For example, certain forms of energy, such as solar or wind, can only be produced under specific conditions (for example, when the sun is up or the wind is blowing). Thus, if you produce this energy at some specific time of the day, and need to use it at another time of the day, it must be put into an energy storage device, or energy accumulator. Energy storage can lead to losses, which in some cases are quite large and in other cases not large at all. In either case the amount of energy produced is less than the amount of energy ultimately available for consumption. This is the so-called intermittancy problem.
Another complication that occurs, is the fact that many countries keep fairly large energy reserves (such as petroleum reserves), that should be worked into maximal energy consumption calculations. For example, existing world fossil fuel reserves could provide several years worth of consumable energy. Thus, the relationship between production and consumption can become quite delicate and very complicated.
That being said, in the long term, assuming ideal storage and transport type conditions, the best case scenario is when production is approximately equal to consumption. So this is the simplification we will take as given, when not explictly stated otherwise. Notice that in general, all realistic assumptions are no better than this. Namely that in order to maintain a certain energy cycle, at least as much or more energy needs to be produced than is ultimately consumed.
Finally, as with the above simplifications, all assumptions below are made under the canopy of trying to attain a collection of “best case scenarios.” What this means is, all assumptions attempt to error on the side of extremely optimistic energy projections, where we set very generous simplifications. The reason to do this, is to show that even under the very best case scenarios, the energy landscape that emerges provides very clear insight into what is meant by energy experts when they refer to our current global energy crisis. That is, though there are many devils in the details, these devils are more than likely trying to distract you from a relatively clear and surprisingly stark larger picture.